Why am I getting old? Molecular Changes Driving Old Age
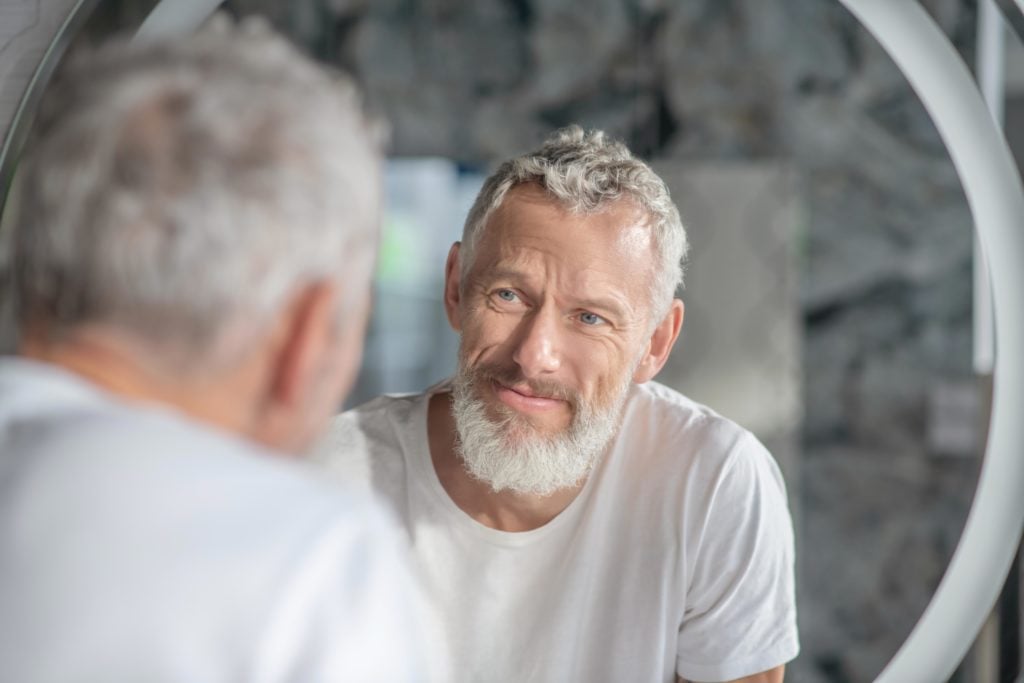
‘Know yourself and know your enemy.
You will be safe in every battle.’
Sun Tzu
Waging War on Frailty
Can we defeat old age?
Sadly, no. At least, not in the immediate future 1.
Can we defeat age-related frailty and disability?
Hell yes!
However, as aging drives frailty, we must first ‘know our enemy’ and develop a deep understanding of the aging process.
Only then can we develop a winning strategy for our war on frailty.
A Quick Biology Primer
DNA
Information is stored within the nucleus in a giant molecule called DNA. Your DNA serves the crucial functions of the long-term storage and processing of the information required for life.
The long DNA molecule is broken into areas called genes, which are the genetic sequences that represent proteins.
Now, your DNA isn’t just floating randomly around within the nucleus, as this quickly leads to tangles because your DNA molecules are so long.
Instead, DNA is carefully wound around globular proteins called histones and then tightly packaged into the molecular structure called chromosomes, which are bundled into the nucleus of your cells (Figure 1) 2.
Imagine that you had to store all your belongings in a small storage unit. You could randomly pack it in, wedge the door shut, and trot off, mission accomplished. But this liaise-a-fair approach creates a serious problem when you need to access high-use objects stuffed behind heavy furniture at the back of the shed.
Obviously, it makes more sense to store your high-use objects at the front, where you can easily access them.
Your cells face a similar challenge. Folding your vast DNA molecules into chromosomes neatly solves the information storage problem. However, it creates a second challenge: how do your cells access genetic information that’s tightly packed within the chromosome?
Epigenetics
The simple answer is epigenetics. Chemical modifications (known as epigenetic changes in the biology jargon) determine whether the DNA is accessible to being translated into protein.
When the DNA-histone complex is open, the DNA can be accessed by protein machinery that reads the DNA. Hence, genes in an open DNA-histone confirmation are highly expressed.
In contrast, enzymes can’t access DNA when tightly bound to histone (i.e., closed), and genes within closed regions aren’t expressed.
Crucially, epigenetics explains why cells such as neurons, muscle cells, and skin cells can have the same genetic material yet completely different shapes, sizes, and biological functions.
For example, while a nerve cell and a muscle cell from the same person have identical genes, the neural and muscle cells have very different epigenetic programs, producing different patterns of protein expression that define their unique biology.
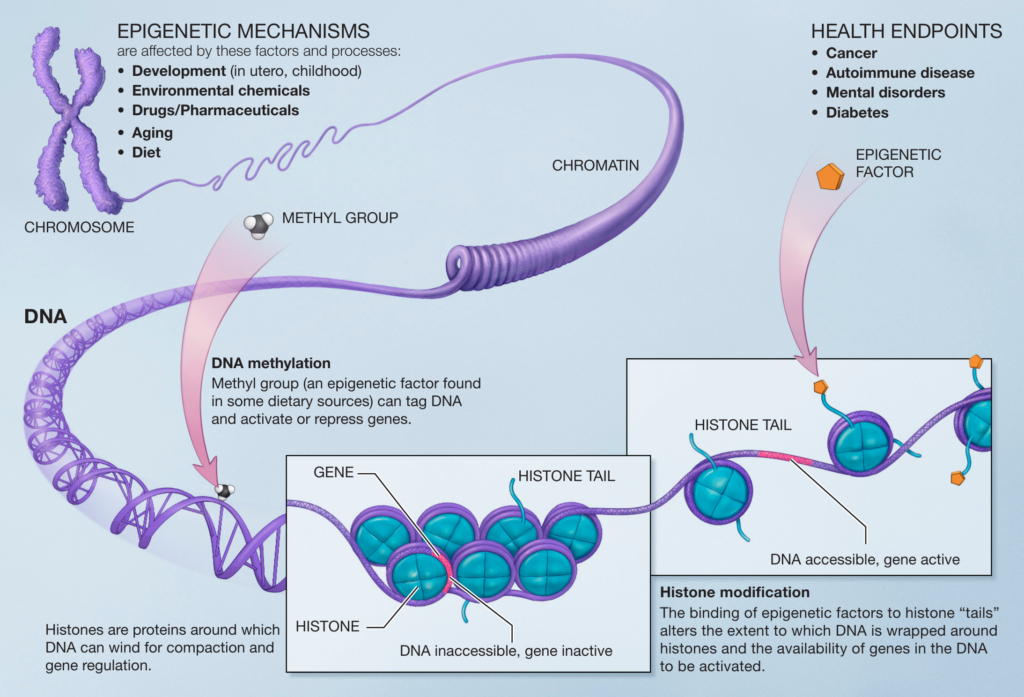
Protein
Protein molecules are the primary workhorses of your cells; they do most jobs that keep you alive. For example,
- Enzymes are proteins that control crucial chemical reactions essential for life.
- Structural proteins provide the shape and strength of your cells.
- Transport proteins move things around.
- Signaling proteins transmit information and form circuits that make cell-level decisions, such as when to divide, move, or die.
- DNA replication and repair? You guessed it; proteins do that too.
The Subcellular Drivers of Aging
1. DNA Mutation and Damage
Although DNA is a proven long-term data storage system, it’s far from perfect.
Indeed, your DNA is constantly under attack by chemical reactions that occur spontaneously within cells 3. Furthermore, exposure to chemicals, radiation, and viruses from the external environment also damages your DNA. For example, smoking exposes the cells of your airways to a host of reactive chemicals that damage your DNA 4.
Because DNA repair is imperfect, repairing these genetic insults often leaves errors 5.
In addition, when a cell divides, it must replicate its entire genome. And while DNA replication is remarkably accurate, rare errors slip through and accumulate over time 5.
Finally, mistakes can be made during cell division, resulting in gains and losses of large chunks of DNA 6.
The constant exposure to genetic insults coupled with imperfect repair and errors during cell division explains why ‘healthy’ cells from young individuals already contain hundreds of mutations.
For example, in human airway cells, an 11-year-old already had 464 mutations per cell, which increased to 2,739 per cell in an 86-year-old individual, giving a mutation accumulation rate of approximately 29 mutations per year 7.
Crucially, mutated genes produce faulty or damaged proteins. As your mutational load increases with age, more proteins become dysfunctional, damaging your cells and compromising the function of physiological systems.
2. Epigenetic Drift
Your DNA is damaged directly through mutation. However, the epigenetic programs that regulate crucial cellular functions and differentiation states degrade over time due to random chemical reactions 8, a process known as epigenetic drift.
Comparing young and old tissue directly reveals dramatic age-related changes in gene-expression patterns caused by epigenetic drift 9.
Crucially, epigenetic gene expression changes negatively impact other aging drivers, such as inflammation, protein folding, extracellular matrix (ECM) regulation, and mitochondrial function, which are widely deregulated in aging 10.
3. Telomere Loss
Telomeres are repetitive DNA sequences found at the end of chromosomes. Their function is to (i) protect the terminal regions of chromosomal DNA from progressive degradation and (ii) prevent DNA-repair mechanisms from joining the ends of chromosomes 11.
However, telomeres aren’t replicated in differentiated cells like neurons and muscles, making them shorter with each cell division. Eventually, as cells divide, the telomeres completely degrade, leading to cell death or senescence 11.
We know that telomeres are essential for aging because telomere shortening decreases the life span of many species 12. Further, mice engineered with longer telomeres enjoy an increased lifespan, improved metabolic health, and reduced cancer incidence 13.
4. Declining Protein Turnover and Quality Control
Like your DNA, your proteins are subject to chemical attacks and accumulate damage over time 14.
To remedy this issue, your cells constantly remove damaged proteins and create new ones to replace them, a process known as protein turnover 15.
However, age-dependent gene mutations and epigenetic drift collaborate to produce erroneously translated, misfolded, or incomplete proteins that collectively overwhelm protein turnover 15. When protein turnover can no longer keep pace, damaged proteins accumulate within your cells, often with catastrophic results.
For example, dreaded neurodegenerative diseases, such as Alzheimer’s, are caused by protein mutations that render them intrinsically prone to misfolding and aggregation 16. Because misfolded and aggregated proteins are particularly resistant to protein turnover, they accumulate within neuronal cells over time, inhibiting cognition and accelerating cognitive decline 17.
5. Mitochondrial Dysfunction and Energy Production
Mitochondria, the ‘powerhouse of the cell,’ are discrete structures within your cells that use aerobic metabolism to produce chemical energy in the form of ATP 18.
Energy production by mitochondria is essential for life, as evidenced by cyanide, which kills by inhibiting mitochondrial function. Organs with high energy demand, such as your brain or heart, are especially vulnerable to mitochondrial decline.
Chemical reactions damage mitochondrial proteins, leading to a gradual but inevitable decline in mitochondrial function. To maintain mitochondrial quality, your cells must constantly replace damaged mitochondria with new ones 18.
Unfortunately, damaged mitochondria accumulate as you age due to reduced mitochondria turnover. These damaged mitochondria produce less energy, enhance the production of damaging reactive oxygen species, and if mitochondria are severely damaged, they can kill your cells 18.
Crucially, mitochondrial dysfunction is linked to many age-related diseases, including Parkinson’s disease, Alzheimer’s disease, cancer, muscle wasting, heart disease, and diabetes 19.
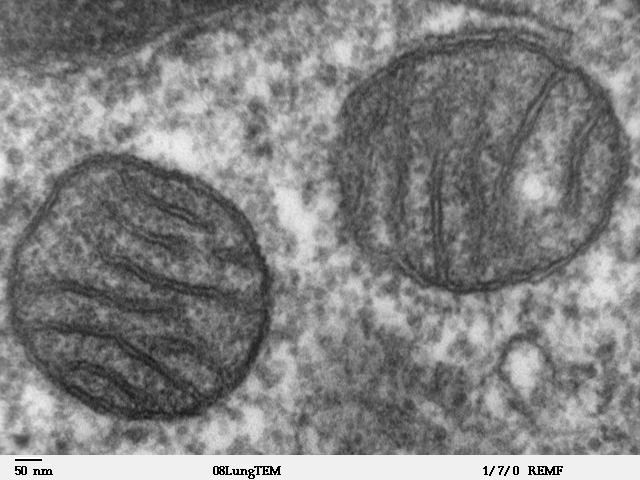
6. Autophagy and Cellular Recycling
Autophagy (from the Greek words auto, meaning ‘self ’, and phagein, meaning ‘to eat’) is a cellular process that plays a crucial role in removing damaged proteins and cellular structures, such as damaged mitochondria 20.
As such, the decline in autophagy is one of the key drivers of aging 21. For example, reducing autophagic flux contributes to accumulating protein aggregates and the accumulation of damaged mitochondria 21. Further, defects in autophagy have been linked to accelerated aging and neurodegenerative disease 21.
Thus, the data from clinical trials, experiments, and studying aging human tissues clearly show that 1) autophagy is crucial for optimal health and longevity, 2) unbalanced autophagy contributes to pathology and disease, and 3) we all experience an age-dependent decline in autophagy 21.
7. Extracellular Matrix Decay
The extracellular matrix (ECM) surrounding your cells is a complex mixture of over 1000 proteins, providing critical structure and support for your cells to flourish 22.
Like the protein inside your cell, ECM proteins are vulnerable to attack from reactive chemicals such as free radicals 22. To compensate for ongoing damage, in most tissues, your ECM undergoes constant turnover to replace the damaged and degraded ECM proteins 22.
Sadly, however, ECM regeneration is not perfect.
For example, cells in your skin deposit collagen into the skin ECM, which provides the support structure that underpins healthy skin and is responsible for preserving skin firmness and elasticity 23. As your skin cells become less active after early adulthood, collagen production declines by about 1.0% a year, causing the wrinkles and saggy skin we all love and cherish 23.
Crucially, ECM decline isn’t limited to your skin. Indeed, age-related ECM declines in all organs, contributing to the loss of organ function, accelerated cellular aging, and age-related disease progression 22.
But wait, aging is complicated!
As you can see, each driver discussed above plays a crucial role in aging. However, aging is far more complicated than simple molecular decay.
For one, the drivers of aging work together to reinforce one another.
For example, DNA mutations can trigger mitochondrial dysfunction, leading to decreased ATP production, which, in turn, exacerbates protein misfolding and autophagic decline.
Conversely, autophagy decline reduces mitochondrial health, creating a feedback loop that increases protein misfolding and disrupts autophagy.
Another potential danger is that many aging drivers serve crucial biological functions, so you can’t just turn them on or switch them off.
For example, while a decline in autophagy certainly contributes to old age and disease, too much autophagy can be equally disastrous.
This was shown in flies (an experimental model used by scientists to understand complex biology), where a mild increase in autophagy increased lifespan. In contrast, a strong increase in autophagy shortened lifespan 24.
Further, cranking up autophagy in humans can have unwanted side effects, such as making cancer more therapy-resistant 25,26.
Take Home Message
We now understand the fundamental drivers of aging, and our knowledge of aging is growing rapidly.
Unfortunately, we now know that aging mechanisms interact within a network of complex feedback loops, leading to increased damage and degeneration over time.
Further, many of the drivers of aging operate within a Goldilocks zone. If you push the drivers of aging too far in either direction, bad things happen.
The million-dollar question is, are we doomed to age out and become frail?
I believe that the answer is a definitive no.
While treating aging is complicated, many studies show that we can delay aging through therapeutic interventions.
Our job moving forward is to optimize anti-aging therapies for maximal benefit and minimal risk.
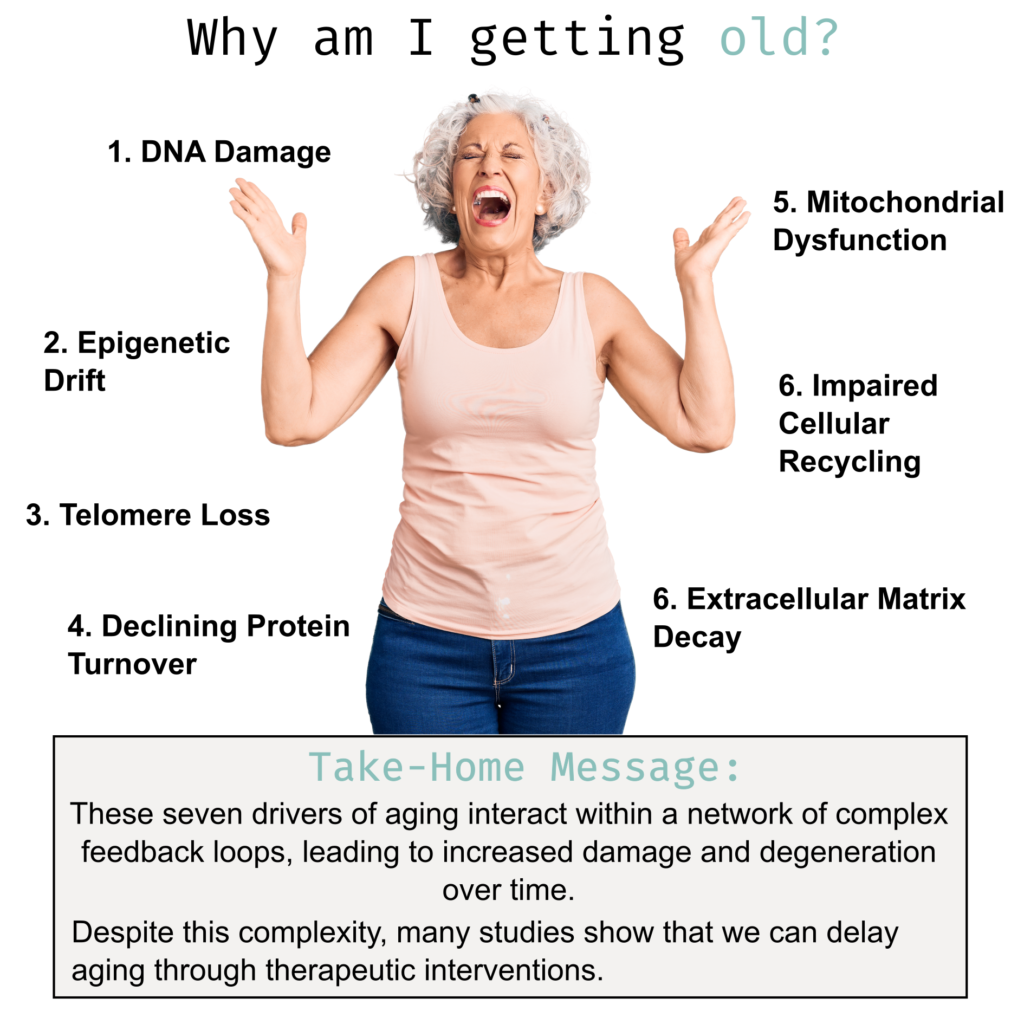
References and Further Reading
1 Milholland, B. & Vijg, J. Why Gilgamesh failed: the mechanistic basis of the limits to human lifespan. Nature Aging 2, 878-884, doi:10.1038/s43587-022-00291-z (2022).
2 Annunziato, A. DNA packaging: nucleosomes and chromatin. Nature Education 1, 26 (2008).
3 Moldogazieva, N. T., Mokhosoev, I. M., Mel’nikova, T. I., Porozov, Y. B. & Terentiev, A. A. Oxidative Stress and Advanced Lipoxidation and Glycation End Products (ALEs and AGEs) in Aging and Age-Related Diseases. Oxid Med Cell Longev 2019, 3085756, doi:10.1155/2019/3085756 (2019).
4 Caliri, A. W., Tommasi, S. & Besaratinia, A. Relationships among smoking, oxidative stress, inflammation, macromolecular damage, and cancer. Mutat Res Rev Mutat Res 787, 108365, doi:10.1016/j.mrrev.2021.108365 (2021).
5 Ren, P., Dong, X. & Vijg, J. Age-related somatic mutation burden in human tissues. Front Aging 3, 1018119, doi:10.3389/fragi.2022.1018119 (2022).
6 Iourov, I. Y., Yurov, Y. B., Vorsanova, S. G. & Kutsev, S. I. Chromosome Instability, Aging and Brain Diseases. Cells 10, doi:10.3390/cells10051256 (2021).
7 Huang, Z. et al. Single-cell analysis of somatic mutations in human bronchial epithelial cells in relation to aging and smoking. Nat Genet 54, 492-498, doi:10.1038/s41588-022-01035-w (2022).
8 Booth, L. N. & Brunet, A. The Aging Epigenome. Mol Cell 62, 728-744, doi:10.1016/j.molcel.2016.05.013 (2016).
9 Hernando-Herraez, I. et al. Ageing affects DNA methylation drift and transcriptional cell-to-cell variability in mouse muscle stem cells. Nat Commun 10, 4361, doi:10.1038/s41467-019-12293-4 (2019).
10 Consortium, T. M. A single-cell transcriptomic atlas characterizes ageing tissues in the mouse. Nature 583, 590-595, doi:10.1038/s41586-020-2496-1 (2020).
11 Blackburn, E. H., Epel, E. S. & Lin, J. Human telomere biology: A contributory and interactive factor in aging, disease risks, and protection. Science 350, 1193-1198, doi:10.1126/science.aab3389 (2015).
12 Whittemore, K., Vera, E., Martínez-Nevado, E., Sanpera, C. & Blasco, M. A. Telomere shortening rate predicts species life span. Proc Natl Acad Sci U S A 116, 15122-15127, doi:10.1073/pnas.1902452116 (2019).
13 Muñoz-Lorente, M. A., Cano-Martin, A. C. & Blasco, M. A. Mice with hyper-long telomeres show less metabolic aging and longer lifespans. Nat Commun 10, 4723, doi:10.1038/s41467-019-12664-x (2019).
14 Nowotny, K., Jung, T., Grune, T. & Höhn, A. Accumulation of modified proteins and aggregate formation in aging. Exp Gerontol 57, 122-131, doi:10.1016/j.exger.2014.05.016 (2014).
15 Hipp, M. S., Kasturi, P. & Hartl, F. U. The proteostasis network and its decline in ageing. Nat Rev Mol Cell Biol 20, 421-435, doi:10.1038/s41580-019-0101-y (2019).
16 Danial, J. S. H. & Klenerman, D. Single molecule imaging of protein aggregation in Dementia: Methods, insights and prospects. Neurobiol Dis 153, 105327, doi:10.1016/j.nbd.2021.105327 (2021).
17 Balusu, S., Praschberger, R., Lauwers, E., De Strooper, B. & Verstreken, P. Neurodegeneration cell per cell. Neuron, doi:10.1016/j.neuron.2023.01.016 (2023).
18 Amorim, J. A. et al. Mitochondrial and metabolic dysfunction in ageing and age-related diseases. Nat Rev Endocrinol 18, 243-258, doi:10.1038/s41574-021-00626-7 (2022).
19 Chocron, E. S., Munkácsy, E. & Pickering, A. M. Cause or casualty: The role of mitochondrial DNA in aging and age-associated disease. Biochimica et Biophysica Acta (BBA) – Molecular Basis of Disease 1865, 285-297, doi:https://doi.org/10.1016/j.bbadis.2018.09.035 (2019).
20 Levine, B. & Kroemer, G. Biological Functions of Autophagy Genes: A Disease Perspective. Cell 176, 11-42, doi:10.1016/j.cell.2018.09.048 (2019).
21 Aman, Y. et al. Autophagy in healthy aging and disease. Nat Aging 1, 634-650, doi:10.1038/s43587-021-00098-4 (2021).
22 Ewald, C. Y. The Matrisome during Aging and Longevity: A Systems-Level Approach toward Defining Matreotypes Promoting Healthy Aging. Gerontology 66, 266-274, doi:10.1159/000504295 (2020).
23 Reilly, D. M. & Lozano, J. Skin collagen through the lifestages: importance for skin health and beauty. Plastic and Aesthetic Research 8, 2 (2021).
24 Bjedov, I. et al. Fine-tuning autophagy maximises lifespan and is associated with changes in mitochondrial gene expression in Drosophila. PLoS Genet 16, e1009083, doi:10.1371/journal.pgen.1009083 (2020).
25 Das, C. K., Mandal, M. & Kögel, D. Pro-survival autophagy and cancer cell resistance to therapy. Cancer Metastasis Rev 37, 749-766, doi:10.1007/s10555-018-9727-z (2018).
26 Mulcahy Levy, J. M. & Thorburn, A. Autophagy in cancer: moving from understanding mechanism to improving therapy responses in patients. Cell Death Differ 27, 843-857, doi:10.1038/s41418-019-0474-7 (2020).
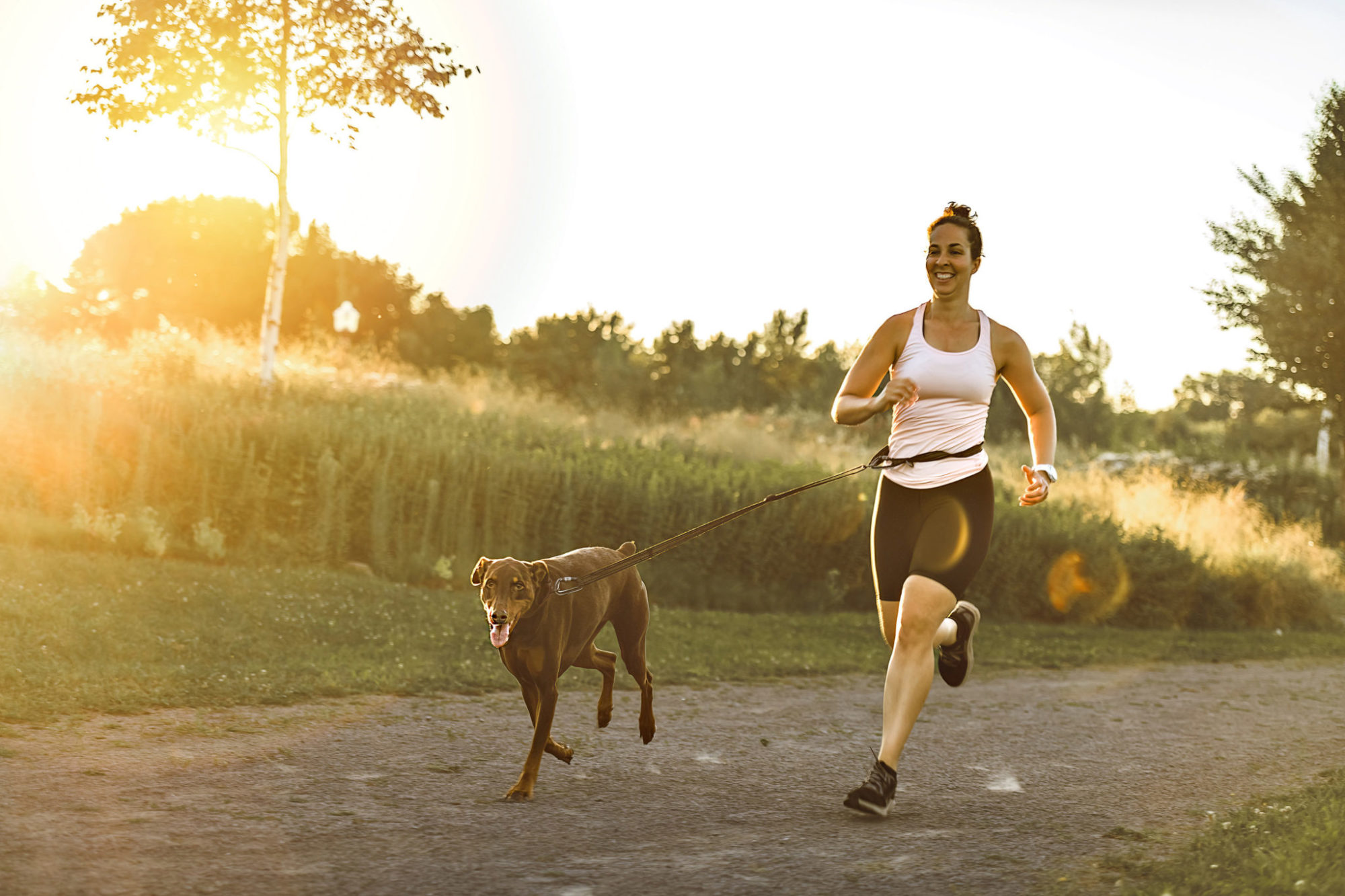
Ten Minutes is All You Need
Research has shown that ten minutes of moderate-to-vigorous exercise performed each day is enough to significantly reduce your risk of early death.
- « Previous
- 1
- …
- 31
- 32
- 33